Master Thesis Proposals
The EO-group offers several thesis projects for both students of the fully integrated master's program (30sp) and the 2-year master's program in Physics (60sp).
Thesis projects are supervised by our staff members and are directly related to the work we are doing in the group.
We gladly welcome student suggestions for projects in any topic related to earth observation. Please contact our staff members to discuss your project ideas.
If you do not have a specific topic in mind, feel free to look at the list of proposed projects here.
The Earth Observation group currently proposes the following projects:
- Tracking the seasonal evolution of radar backscatter signatures from different sea ice types
- Summer sea ice density from multi-sensor airborne data
- Is the Arctic sea ice becoming more hostile to radar altimetry?
- Ice berg detection using SAR
- Sea ice vs open water in Svalbard fjords
- Multi-frequency SAR study of thinner sea ice and ridged areas
- Thin sea ice detection with CryoSat-2
- SAR polarimetric sea ice evolution during the CIRFA 2022 cruise
- Can cold snow interfere with radar signals?
- Influence of height, distance, shape, and orientation of pressure ridges on the X-band ice ship radar
- Naturally occurring oil seeps in Hopendjupet and how to model their drift patterns
If you are interested in doing a project with us, please send us an e-mail, or stop by our offices.
For examples of earlier completed master projects, see the Education section.
Tracking the seasonal evolution of radar backscatter signatures from different sea ice types
Synthetic aperture radar (SAR) is the main tool for operational sea ice monitoring. Due to its all-day and all-weather imaging capability, SAR instruments can reliably provide images throughout the entire year. The radar signal that is backscattered from the sea ice and snow surface is controlled by both surface and radar parameters. The most important radar parameters are frequency, polarization, and incident angle (IA), the dominant surface parameters include small-scale roughness (on the scale of mm to dm), large-scale roughness (deformation on the scale of dm to m), and the electric properties of the ice and snow.
For a given sea ice type, such as for example level first-year ice (FYI) or deformed multi-year ice (MYI), variations in IA and changes in the overlying snowpack during melt onset can cause large variations in the radar backscatter. In the past, these variations have mostly been investigated for stationary landfast ice. In this master project, you will study the effect of IA and seasonal changes on drifting sea ice.
During the CIRFA-22 cruise in April and May 2022, we deployed 17 drift buoys (drifters) on the sea ice close to Greenland. The drifters sampled GPS position every 30 minutes, resulting in the drift trajectories shown in the left figure. These trajectories enable us to identify and track individual sea ice floes in a time series of overlapping Sentinel-1 SAR images and track their backscatter signature over the course of several weeks and months. The figure on the right shows an example result of HH and HV intensity time series from a MYI and an FYI floe, indicating the manually identified timing of melt onset. In this master thesis, you will use the drifter data and overlapping Sentinel-1 imagery to expand the amount of tracked ice floes, and you will identify additional ice types in the imagery and include them in the study. You will then calculate the statistical separability of the different ice types and use this to assess the results of an automated ice type classification algorithm. Finally, you will extract backscatter signature time series over the landfast ice on the Greenland coast and investigate the differences and similarities in the evolution of drift ice and landfast ice backscatter. Depending on your initial findings, progress, and personal interest, there is also the option to work on retraining the classifier to enhance its performance during the melt season.
During this project, you will learn about sea ice types, drift, and classification, and you will gain experience in SAR image processing and interpretation. The work is directly related to several ongoing projects in the Earth Observation group, and you will have a chance to closely work with our PhD students or post-docs.
Left: Trajectories of drifters deployed on the sea ice during the CIRFA-22 cruise in April and May 2022 (map by C. Taelman). Photograph of a drifter after its deployment in the top left corner. Right: Time series of IA-corrected backscatter from example MYI and FYI floes (from Taelman et al., 2023).
Contact: Johannes (johannes.p.lohse@uit.no), Catherine (catherine.c.taelman@uit.no)
Literature:
Taelman, C., Lohse, J., Doulgeris, A.P., Johansson, M. (2023). From winter to melt season: C-band radar backscatter evolution of fast-drifting sea ice floes. Poster presentation at IGS 2023. 10.13140/RG.2.2.11564.44164
Lohse, J., Doulgeris, A.P., Dierking, W. (2020). Mapping sea-ice types from Sentinel-1 considering the surface-type dependent effect of incidence angle. Annals of Glaciology, 61(83), 260-270.
https://doi.org/10.1017/aog.2020.45
Geldsetzer T., Howell, S. (2023). Incidence angle dependencies for C-band backscatter from sea ice during both the winter and melt season. IEEE Transactions on Geoscience and Remote Sensing. 10.1109/TGRS.2023.3315056
Summer sea ice density from multi-sensor airborne data
One of the largest outstanding uncertainties in state-of-the-art polar sea ice thickness datasets is the sea ice density. Satellite altimeters measure the ice freeboard, which must be converted to the ice thickness with an assumption about the ice density. So far, these assumptions have been ultra-simple, with a single ice density assumed for different sea ice types.
In this project we will work with the Alfred Wegener Institute (AWI) who have collected multi-sensor airborne datasets on their IceBird campaigns in the Arctic to estimate the sea ice density and re-evaluate the assumptions made for satellite ice thickness products. This has been tested for data in winter by Jultila et al (2022) https://tc.copernicus.org/articles/16/259/2022/ but here we will use data from the laser scanner and "EM-bird" to produce unique measurements of sea ice density in the Arctic summer.
Contact: Jack Landy (jack.c.landy@uit.no)
Is the Arctic sea ice becoming more hostile to radar altimetry?
Sea ice thickness is estimated using radar-satellites by measuring how far the floating ice sticks out of the water; this involves measuring the elevation of the floating ice surface, and the elevation of nearby open water. The difference is known as the "ice freeboard". The technique requires the "clean" reflection of radar pulses from one surface type: ice or water. It also helps when the ice surface is floating further above the waterline, with freeboards
Arctic sea ice is becoming thinner and more mobile as the atmosphere warms, and these changes will continue over the 21st Century. This raises the question of if, and when, Arctic freeboards will become too small to measure with existing approaches. So what is the future of freeboard in the Arctic Ocean? This project will analyse sea ice and snow thickness data in projections from the latest generation of climate models to determine how Arctic sea ice freeboard will evolve over the 21st Century.
Because the sea ice is also becoming more mobile, gaps in the sea ice called "leads" are becoming increasingly common. This raises the question of whether it's becoming harder to reflect radar from pulses sea ice floes alone, without contamination from nearby water. To assess the future of this challenge, this project will also analyse radar data from the CryoSat-2 altimeter from different sea ice thicknesses and types. We know the "ice of the future" is younger and thinner, so is this ice harder to measure than the existing older, thicker ice?
The future of radar altimetry in the Arctic is bound up in the future of sea ice freeboard and mobility.
Your results will be relevant to the long-term planning and strategy of Earth-Observing organisations such as the European Space Agency and NASA.
Contact: Robbie Mallet (robbie.d.mallett@uit.no)
Ice berg detection using SAR
Primary Objective: To compare very high-resolution commercial SAR data with Copernicus Sentinel-1 data to determine the minimum viable iceberg size detectable with Sentinel-1.
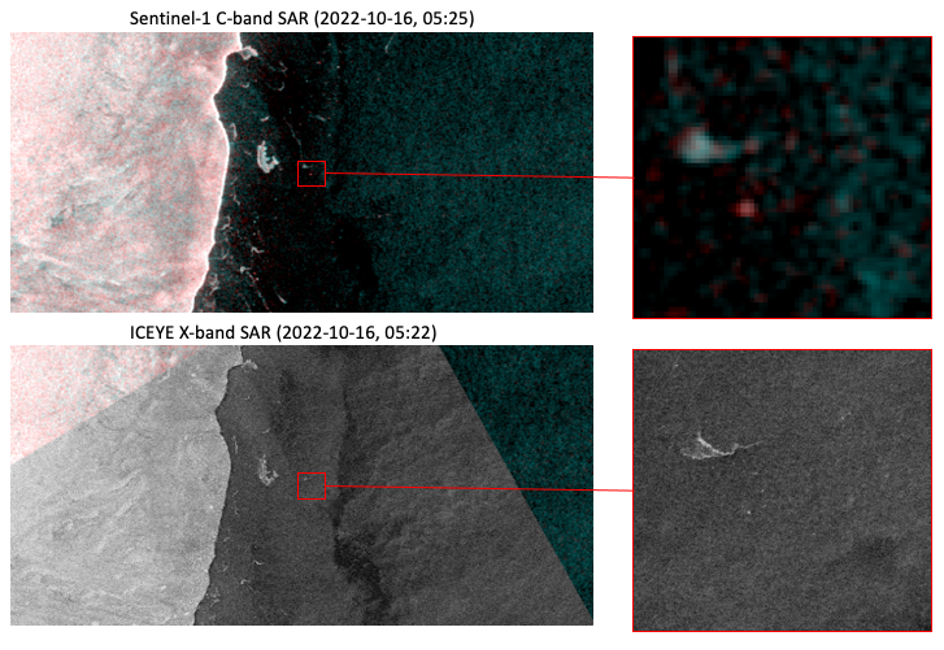
Data
Contact: Anthony Doulgeris (anthony.p.doulgeris@uit.no)
Sea ice vs open water in Svalbard fjords
Separation between sea ice and open water in fjords in Svalbard is challenging. Wind conditions are ever changing, the sea ice is forming in-situ and is drifting.
We have established a time series starting in 2014 with Sentinel-1 data for three fjords on Svalbard, Hornsund, Kongsfjorden and Rijpfjorden.
The goal here is to train a neural network into automatically separating open water from sea ice. Though the challenging wind conditions and the low signal of thin nice means that some images had to be removed due to poor quality. It's important that an AI based system can learn why these images were considered poor.
Alternatively can the project focus on the melting snow cover on the sea ice. Information about wet snow cover on shore (from the same Sentinel-1 time series https://doi.org/10.3389/feart.2022.868945 ) exist and be used as a proxy to derive melting snow on the sea ice.
Contact: Malin Johansson (malin.johansson@uit.no)
Multi-frequency SAR study of thinner sea ice and ridged areas
The Arctic sea ice landscape is rapidly transforming and this includes a thinning of the sea ice and a reduction of the sea ice extent. The dark and often cloudy environment means that satellite-borne radar sensors, with multi-polarization capabilities, play a key role in sea ice monitoring. The marginal ice zone in particular is subjected to cloud cover and fog and is also an area of specific interest for guiding ship traffic and for the maritime industry. Ice regimes differ in composition and areal fractions of ice types, ice concentration, and degree of deformation.
The image shows thin sea ice that has undergone finger rafting. Image taken during the CIRFA cruise in April-May 2022
C-band SAR is used operationally to map the presence of sea ice as well as classify different sea ice types. However, L-band SAR has been shown to be sensitive to deformed sea ice areas, and in addition, the good signal-to-noise ratio at L-band enables good separation of young ice types from surrounding thicker sea ice and the open water. The larger penetration depth in L-band SAR is of general benefit for characterizing and classifying sea ice, as it improves discrimination between first (FYI) and multiyear ice (MYI), and is of specific benefit for detection and characterization of leads and thin ice areas. The low noise floor for ALOS-2 enables reliable separation of thin ice areas from thick sea ice and also in the presence of frostflowers.
This project will build upon overlapping fully polarimetric L- and C-band data collected during the MOSAiC campaign in 2019-2020 and during the CIRFA cruise in 2022 to Belgica Bank. The aim is to investigate the importance of pixel resolution for the detection of thin ice and ridges and how this varies with the two different frequencies. This can be done using both spatially and temporally overlapping medium-resolution images such as those from the Sentinel-1 or by simulating images with a lower resolution. The outcome is expected to provide answers to the size of thin ice areas and ridges that can be captured in the high-resolution fully polarimetric images and how this translates to the daily medium-resolution Sentinel-1 images.
Contact: Malin Johansson (malin.johansson@uit.no)
SAR polarimetric sea ice evolution during the CIRFA 2022 cruise
In April – May 2022 CIRFA conducted a cruise to the Belgica bank area. During this cruise, in-situ data was collected to confirm sea ice types and other sea and snow surface parameters that we can observe in the satellite images. Sensors were installed on the sea ice to keep recording temperature data even after the cruise. Regular fully-polarimetric C- and L-band SAR images were collected over this area until the end of June.
Pauli images from April 11 (before the cruise), May 4 (under the cruise), and June 18 (after the cruise). The images cover in part the same area.
The data set in this project consists of primarily 11 Radarsat-2 scenes collected over fast ice in the western Fram Strait in the period April 11th to June 18th, 2022. The scenes cover several types of snow-covered sea ice areas, including level first-year ice, deformed multi-year ice, and thin young lead ice. In the first part of the time period, the area had cold winter conditions, with dry snow cover. The temperatures gradually increased through the last part of May, and towards the end of the data collection, the site area experienced warm temperatures and melting conditions. As can be seen, different ice surfaces undergo different changes, which we think are correlated to the physical structure of the surfaces.
Within this project the backscatter evolution as well as the evolution of polarimetric features from the winter season until the melt season will be investigated. The effect of the onset of melt season on different sea ice types, such as smooth and deformed, will be analyzed and if possible, connected to scattering models.
Contact: Malin Johansson (malin.johansson@uit.no)
Can cold snow interfere with radar signals?
It is normally assumed that the cold snow layer is transparent to the light in the microwave part of the spectrum. The actively sent pulse of light should scatter predominantly on the sea ice surface or shortly after penetrating it. Recent research shows that that does not hold for salty and wet snow (Nandan et al, 2017). This knowledge is essential for correct retrieval of sea ice thickness and snow from radar altimeters mounted on satellites (e.g. CryoSat-2, Sentinel-6, and future ESA missions). At MOSAiC several thousand snow depth measurements were collected each week along several-kilometer-long transects. At the same location, sea ice thickness was recorded. The X-band ice radar mounted on the research ship (Oikkonen et al, 2017) was recorded continuously over large areas surrounding those measurements. The first look at the imagery suggests that large artificial snow accumulations can change X-band radar intensities. While the Arctic snow cover is typically thin (10-30 cm), large amounts of snow can accumulate also in naturally-occurring features of pack ice -in the pressure ridges (Liston et al, 2018). When the ridges are first formed the snow cover there is the same thin as everywhere else and even blocks of bare ice are exposed. Over time, ridges as rough features in windy environments accumulate deep snow drifts, in places over 1m deep. For this master thesis topic, the candidate will explore the development of ship radar back-scatter intensities and compare them to the snow depth observed in situ on the ice. The candidate should be interested in sea ice geophysical processes and statistical analysis of the data. The analysis could be potentially extended to satellite radar data and radar signal modeling.
Literature:
- Nandan, V., Geldsetzer, T., Yackel, J., Mahmud, M., Scharien, R., Howell, S., ... Else, B. (2017). Effect of snow salinity on CryoSat‐2 Arctic first‐year sea ice freeboard measurements. Geophysical Research Letters, 44, 10,419–10,426. https://doi.org/10.1002/2017GL074506
- Oikkonen, A., Haapala, J., Lensu, M., Karvonen, J., andItkin, P. (2017), Small‐scale sea ice deformation during N‐ICE2015: From compact pack ice to marginal ice zone, J. Geophys. Res. Oceans, 122, 5105–5120, doi:10.1002/2016JC012387.
- Liston, G. E., Polashenski, C., Rösel, A., Itkin, P., King, J., Merkouriadi, I., & Haapala, J. (2018). A distributed snow‐evolution model for sea‐ice applications (SnowModel). Journal of Geophysical Research: Oceans, 123, 3786‐ 3810. https://doi.org/10.1002/2017JC013706
Contact: Anthony Doulgeris (anthony.p.doulgeris@uit.no)
Influence of height, distance, shape, and orientation of pressure ridges on the X-band ice ship radar
The images from navigational sea ice ship radar could be used to develop sea ice classification (e.g. see pioneering work with ship radar by Kaspersen (2017), and sea ice classification examples in Lohse et al, 2020). The images with radar intensities can be compared to airborne data: Airborne Laser Scanner (ALS) from the helicopter on MOSAiC expedition (for example data from the previous campaign see: Itkin et al, 2018). Some data is available as ‘raw’ radar backscatter, but the majority of the data is already converted to signal intensity. How can we best use such limited data? Additional information that can be used is in-situ data from ice cores and snow pits inside the radar footprint, ice observations from the ship, and back trajectory analysis of SAR images and passive microwave products. The candidate should be interested in image analysis, sea ice geophysical processes, and statistical analysis of the data. The topic has the potential to develop into a PhD thesis.
Literature:
- Kaspersen K. M., 2017, Master Thesis at UiT
- Lohse J, Doulgeris AP,Dierking W (2020). Mapping sea-ice types from Sentinel-1 considering the surface-type dependent effect of incidence angle. Annals of Glaciology1–11. https://doi.org/10.1017/aog.2020.45
- Itkin, P., Spreen, G., Hvidegaard, S. M., Skourup, H., Wilkinson, J., Gerland, S., & Granskog, M. A. (2018). Contribution of deformation to sea ice mass balance: A case study from an N‐ICE2015 storm. Geophysical Research Letters, 45, 789–796. https://doi.org/10.1002/2017GL076056
- Cruise reports from MOSAiC expedition (in preparation, drafts available as internal material on request from polona.itkin@uit.no)
- Cruise report from Nansen Legacy cruise SSQ2 –April/May 2021 (draft available in June 2021)
Contact: Anthony Doulgeris (anthony.p.doulgeris@uit.no)
Naturally occurring oil seeps in Hopendjupet and how to model their drift patterns
In the Barents Sea hydrocarbons (oil and natural gas) are stored in the seabed, and methane gas and oil is naturally seeping out and rising to the ocean surface. Known hot spots for such oil releases are Hopendjupet and the area outside Prins Karls Forland. These oil seepages can be observed in synthetic aperture radar (SAR) images.
It's common that these slicks have a drift pattern that has the shape of spirals (see figure). These spirals are partially caused by inertial oscillations. The frequency of these depends (to first order) on the latitude and to second order on the stratification. The frequency is possible to derive from, e.g., the Norwegian Norkyst-800 or Barents 2.5 model. The amplitude however will be inferred from the SAR images and within this project it’ll be investigated how well the amplitude of the near-intertial waves is resolved in the models.
Radarsat-2 image (left) with naturally occurring seepages shown as dark spirals, and Sentinel-1 SAR image (right) with overlayed areas showing the slick detections during one week.
The supervision will be shared between UiT and the Meteorological Institute.
Contact: Malin Johansson (malin.johansson@uit.no) or Johannes Röhrs (johannesro@met.no)