METHANE IN THE ARCTIC BIOGEOSPHERE
THE ARCTIC OCEAN IS A CRITICAL REGION IN A CHANGING GLOBAL CLIMATE
The Arctic is particularly vulnerable to rising temperatures in ocean and atmosphere. Methane hydrate stability may impact many of the interconnected processes - biological, chemical, geological - in the Arctic ocean.
Methane Hydrates are crystalline compounds which are found in marine and permafrost regions world-wide. Hydrate is generally stable in the subsurface under specific temperature and pressure regimes, however as the arctic ocean warms, gas hydrate destabilization can occur. Destabilization of gas hydrate leads to methane release onto the seabed and into the water column. There are microbes which live in the seabed and in the water column that can consume methane, however if methane reaches the atmosphere it acts to warm as a potent greenhouse gas, keeping warmth trapped in the atmosphere and contributing to a warming climate.
It is crucial that we understand how methane affects coupled biological, chemical and geologic processes, especially in the Arctic Ocean.
AKMA supports students to undergo the training and gain knowledge to become the future Arctic Oceanographic experts.
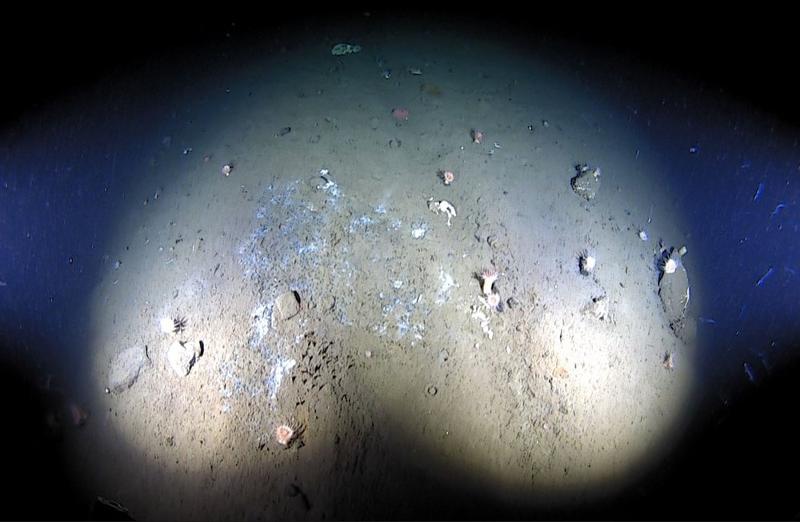
SVYATOGOR RIDGE
The Svyatogor Ridge is the sedimented inside corner high of the Knipovich Ridge-Molloy Transform Fault intersection. The Knipovich Ridge is an ultra slow, melt poor spreading ridge that is part of the Arctic mid ocean ridge system. Typically spreading on melt poor spreading ridges is accommodated by detachment faulting where material from the mantle is exhumed to create the new oceanic crust. The inside corner high is typically a bathymetric high due to a combination of the detachment faulting, the stress regimes between the spreading ridge and transform fault and the thermal profile of the crust at these intersections. When the mantle material exhumed along the detachment faults is exposed to water, it can undergo a metamorphosis called serpentinization. Following serpentinization along detachment faults at spreading ridges, methane from some of the products of serpentinization can form. This methane does not require the input of organic matter to form, so is called abiotic methane, i.e. not organic in origin.
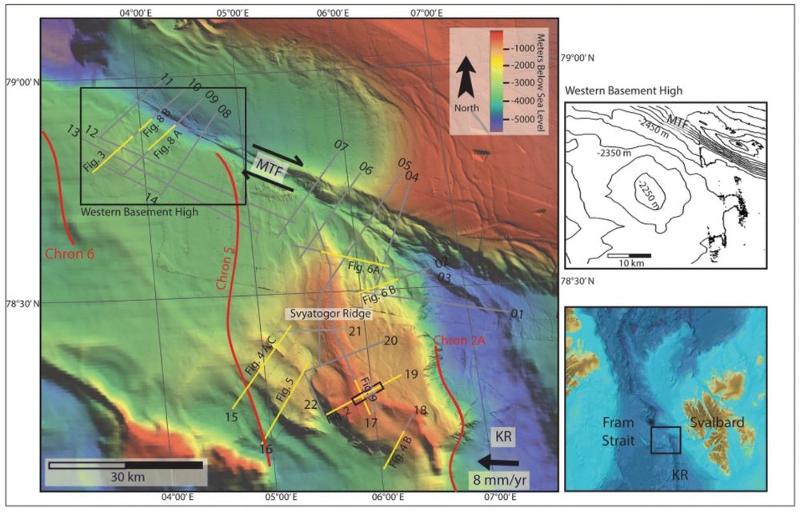
This type of geologic setting would normally lend itself to a hydrothermal vent fluid flow system because it’s a mid ocean ridge, temperatures tend to be fairly high and mid ocean ridges are, well, in the middle of the ocean. Sedimentation in the depths of the ocean is typically minimal and coupled with a constantly moving crust, these types of environments don’t really accumulate all that much sediment. However, this site is a little different. It is very close to the West Svalbard Margin and there is a major oceanic current, the West Spitsbergen Current, which flows along the West Svalbard Margin. This current brings sediment north and reworks, deposits and erodes sediment along the way. Over the last couple million years, the Svyatogor Ridge has also been proximal to another major sediment source, the sediment brought offshore by glaciers from Svalbard. So, the Svyatogor Ridge, and generally this section of the Knipovich Ridge has a lot more sediment than many other spreading ridges and inside corner highs. So, any fluid that moves around in this system can be ‘caught’ by the sediment. The sediment might also act to insulate the crust, so temperatures at the seafloor may be a bit lower than expected, while delaying the cooling process at the interface between the crust and the sediment. And, instead of a hydrothermal vent system typical for mid ocean spreading ridges we actually see a gas hydrate system. Various publications speculate on whether there might be abiotic methane contributing to this gas hydrate.
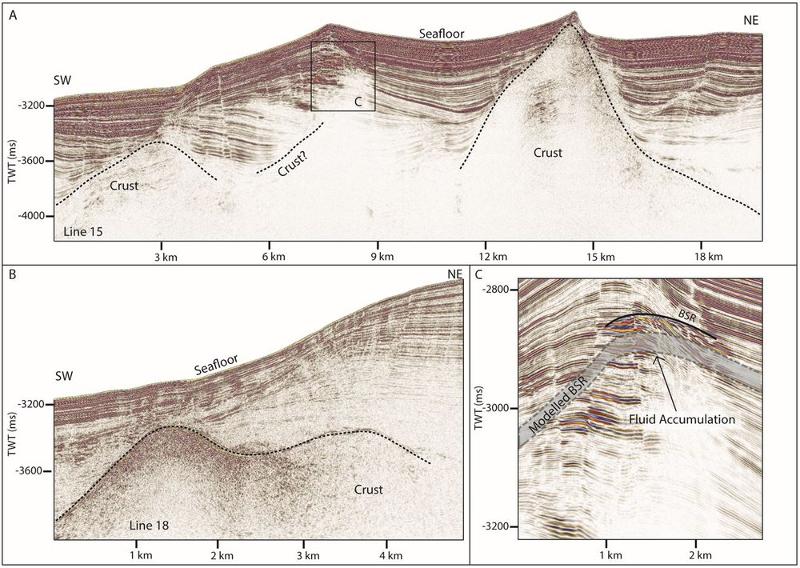
The Svyatogor Ridge has evidence of a gas hydrate system, and our expedition in 2021 found an ecosystem in relation to the Svyatogor Ridge where we confirmed that there is methane reaching the seafloor here. Now the questions we want to answer relate to how this quite unique ecosystem may be different or similar to hydrothermal vents or cold seeps in other Arctic fluid flow systems, and whether this type of system might be more prevalent along the Arctic mid ocean spreading ridge system.
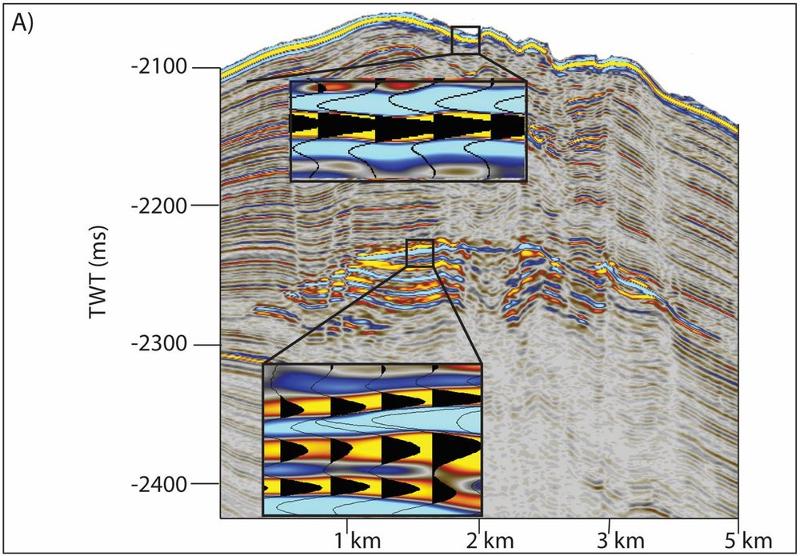
Related publications:
Crane, K., Sundvor, E., Buck, R. & Martinez, F. (1991). Rifting in the northern Norwegian-Greenland Sea: Thermal tests of asymmetric spreading. Journal of Geophysical Research: Solid Earth. 96, 14529-14550.
Eiken, O. & Hinz, K. (1993). Contourites in the Fram Strait. Sedimentary Geology. 82, 15–32.
Ehlers, B.M. & Jokat, W. (2009). Subsidence and crustal roughness of ultra-slow spreading ridges in the northern North Atlantic and the Arctic Ocean. Geophysical Journal International. 177(2), 451-462
Himmler, T., Sahy, D., Martma, T., Bohrmann, G., Plaza-Faverola, A., Bünz, S., Condon, D. J., Knies, J. & Lepland, A. (2019). A 160,000 year-old history of tectonically controlled methane seepage in the Arctic. Science Advances. EAAW1450
Howe, J.A., Shimmield, T.M., Harland, R.E.X., Eyles, N. (2008). Late Quaternary contourites and glaciomarine sedimentation in the Fram Strait. Sedimentology 55, 179–200.
Johnson, J.E., Mienert, J., Plaza-Faverola, A., Vadakkepuliyambatta, S., Knies, J., Bünz, S., Andreassen, K. & Ferre, B. (2015) Abiotic methane from ultraslow-spreading ridges can charge Arctic gas hydrates. Geology, 43(5), 371-374.
Pape, Thomas; Buenz, Stefan; Hong, Wei-Li; Torres, Marta E.; Riedel, Matthias; Panieri, Giuliana; Lepland, Aivo; Hsu, Chieh Wei; Wintersteller, Paul; Wallmann, Klaus; Schmidt, Christopher; Yao, Haoyi; Bohrmann, Gerhard, 2019. Origin and transformation of light hydrocarbons ascending at an active pockmark on Vestnesa Ridge, Arctic Ocean. Journal of Geophysical Research (JGR): Solid Earth. ISSN: 2169-9313, e-ISSN: 2169-9356,
Ritzmann, O., Jokat, W., Mjelde, R., & Shimamura, H. (2002). Crustal structure between the Knipovich Ridge and the Van Mijenfjorden (Svalbard). Marine Geophysical Researches, 23(5-6), 379-401.
Waghorn, K. A., Bünz, S., Plaza‐Faverola, A., & Johnson, J. E. (2018). 3‐D Seismic Investigation of a Gas Hydrate and Fluid Flow System on an Active Mid‐Ocean Ridge; Svyatogor Ridge, Fram Strait. Geochemistry, Geophysics, Geosystems, 19(8), 2325-2341.
Waghorn, K.A., Vadakkepuliyambatta, S., Plaza-Faverola, A., Johnson, J.E., Bünz, S. & Waage, M. (2020). Crustal processes sustain Arctic abiotic gas hydrate and fluid flow systems.
HÅKON MOSBY MUD VOLCANO
The Håkon Mosby Mud Volcano is, as the name suggests, a mud volcano named after Norwegian oceanographer Håkon Mosby. The Håkon Mosby Mud Volcano (HMMV) is located on the Barents Sea Margin at 1250 m water depth. HMMV was discovered in 1990, and scientists aboard the research vessel noted that it was about one square kilometer, and rose only about 10 m from the seafloor. However, the HMMV doesn’t just spew mud, it also emits methane. When the HMMV was discovered, scientists already linked mud volcanism to methane and realized mud volcanoes were important sources of natural methane to both the ocean and the atmosphere. In the case of HMMV, early studies quantified the methane-eating microbes, where they lived around the mud volcano, and importantly the role of the constant mud emissions on the microbial community.
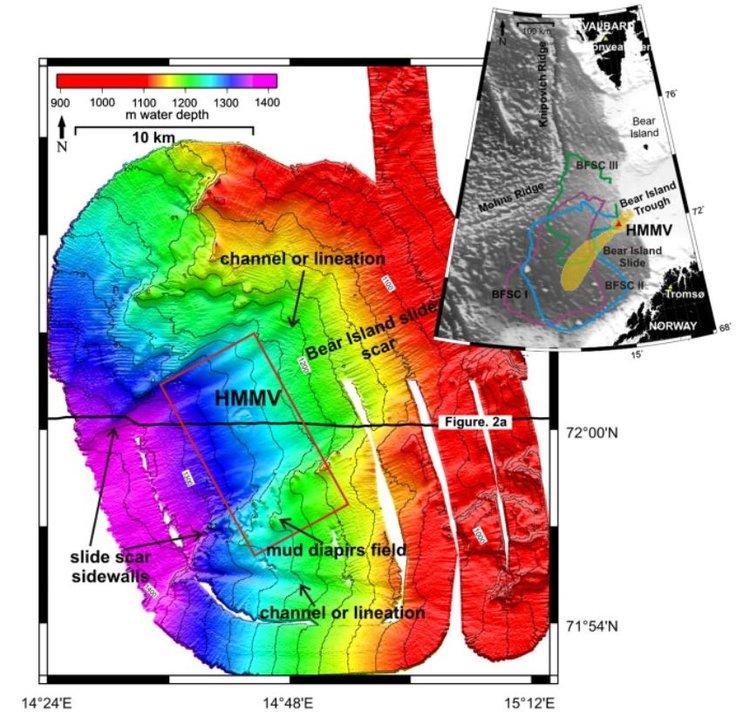
In the early days of research into the HMMV, scientists were not expecting to discover the HMMV where they did. The geologic setting didn’t seem to lend itself towards mud volcanism. A variety of different explanations were possible but some, like igneous processes were ruled out when no intrusions beneath the mud volcano were present. However, the setting is on a formerly glaciated margin in thick glacial sediment deposits that were deposited over a short (geologically short) time frame. We know that there is methane present in the fluids coming from the HMMV, and that they are a mixture of microbial and thermogenic methane. We also know that the HMMV is located in the centre of the Bear Island/Bjørnøya Slide and that studies suggest a link between the submarine slide in the Late Pleistocene and the onset of mud volcanism here. Alternatively, others suggest that mud volcanism started earlier and the slide revitalized the mud volcanism. In any scenario, the exact mechanisms that caused this mud volcano aren’t totally clear yet.
The Håkon Mosby Mud Volcano has been active for many years now. Since it’s discovery it has been active when scientists have visited the site, including our AKMA 1 expedition in 2021 when we were able to use the ROV Ægir to see the mud spewing from crevasses in the mud. Our objectives at this site are mostly geochemical and biological in discipline but from a geological perspective there is still much to understand about how the HMMV formed and the unique location of this particular mud volcano.
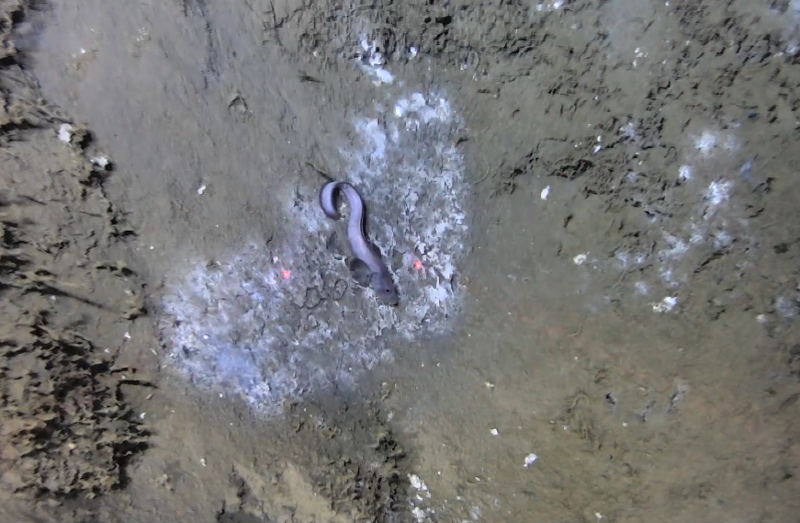
Relevant Research
de Beer, D., Sauter, E., Niemann, H., Kaul, N., Foucher, J. P., Witte, U., ... & Boetius, A. (2006). In situ fluxes and zonation of microbial activity in surface sediments of the Håkon Mosby Mud Volcano. Limnology and Oceanography, 51(3), 1315-1331.
Eldholm, O. S. E. V., Sundvor, E., Vogt, P. R., Hjelstuen, B. O., Crane, K., Nilsen, A. K., & Gladczenko, T. P. (1999). SW Barents Sea continental margin heat flow and Håkon Mosby mud volcano. Geo-Marine Letters, 19(1), 29-37.
Foucher, J. P., Dupré, S., Scalabrin, C., Feseker, T., Harmegnies, F., & Nouzé, H. (2010). Changes in seabed morphology, mud temperature and free gas venting at the Håkon Mosby mud volcano, offshore northern Norway, over the time period 2003–2006. Geo-Marine Letters, 30(3), 157-167.
Hjelstuen, B. O., Eldholm, O., Faleide, J. I., & Vogt, P. R. (1999). Regional setting of Håkon Mosby mud volcano, SW Barents Sea margin. Geo-Marine Letters, 19(1), 22-28.
Jerosch, K., Schlüter, M., Foucher, J. P., Allais, A. G., Klages, M., & Edy, C. (2007). Spatial distribution of mud flows, chemoautotrophic communities, and biogeochemical habitats at Håkon Mosby Mud Volcano. Marine Geology, 243(1-4), 1-17.
Kaul, N., Foucher, J. P., & Heesemann, M. (2006). Estimating mud expulsion rates from temperature measurements on Håkon Mosby Mud Volcano, SW Barents Sea. Marine Geology, 229(1-2), 1-14.
Mienert, J., Dowdeswell, J. A., & Taylor, J. (2003). The Håkon Mosby Mud Volcano. In European Margin Sediment Dynamics (pp. 119-122). Springer, Berlin, Heidelberg.
Milkov, A. V., Vogt, P. R., Crane, K., Lein, A. Y., Sassen, R., & Cherkashev, G. A. (2004). Geological, geochemical, and microbial processes at the hydrate-bearing Håkon Mosby mud volcano: a review. Chemical Geology, 205(3-4), 347-366.
Niemann, H., Lösekann, T., De Beer, D., Elvert, M., Nadalig, T., Knittel, K., ... & Boetius, A. (2006). Novel microbial communities of the Haakon Mosby mud volcano and their role as a methane sink. Nature, 443(7113), 854-858.
Perez-Garcia, C., Feseker, T., Mienert, J., & Berndt, C. (2009). The Håkon Mosby mud volcano: 330 000 years of focused fluid flow activity at the SW Barents Sea slope. Marine Geology, 262(1-4), 105-115.
The Vestnesa Ridge is an elongated sediment drift offshore West Svalbard. It bends from N-S to NW-SE, and all the pockmarks are located along the crest of the ridge.
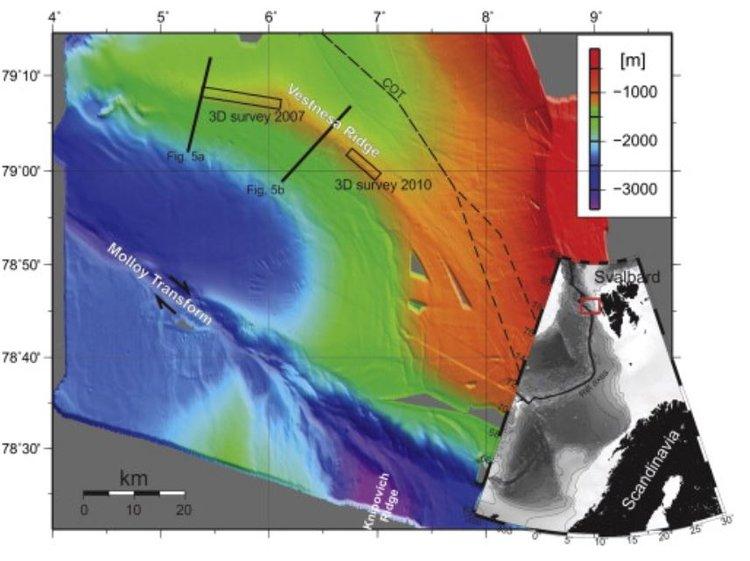
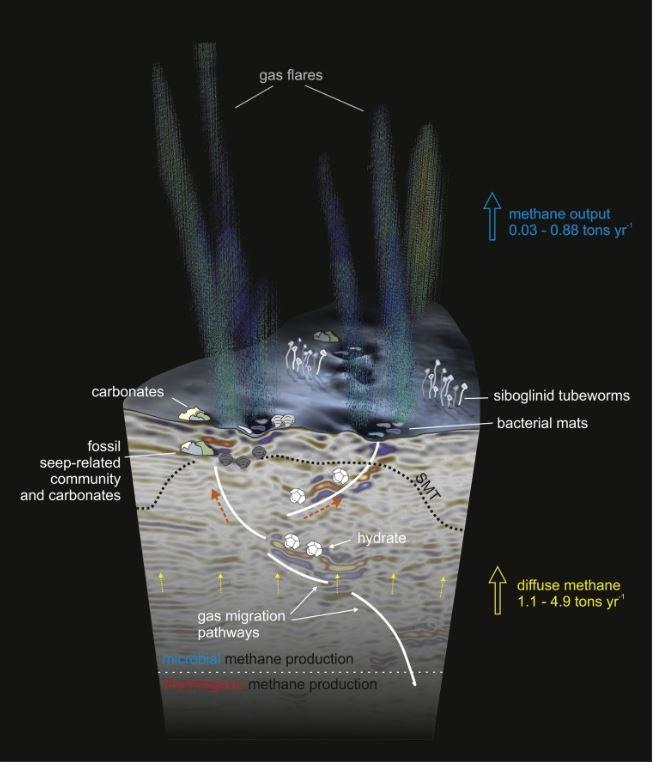
The pockmarks on the seafloor are large - they are around 700 m in diameter and about 10 m deep. The pockmarks of Vestnesa Ridge are home to carbonate blocks, chemosynthetic communities, gas hydrates and smaller (about 50 m wide) pits. The carbonate blocks reach around 3 m high and are evidence that much of the carbon released at the site is not reaching the ocean. ‘Bright spots’ in the subsurface, on seismic data, close to the pockmarks and pits are thought to be fossil seep related communities, communities and free gas accumulations providing evidence for long term methane seepage here.
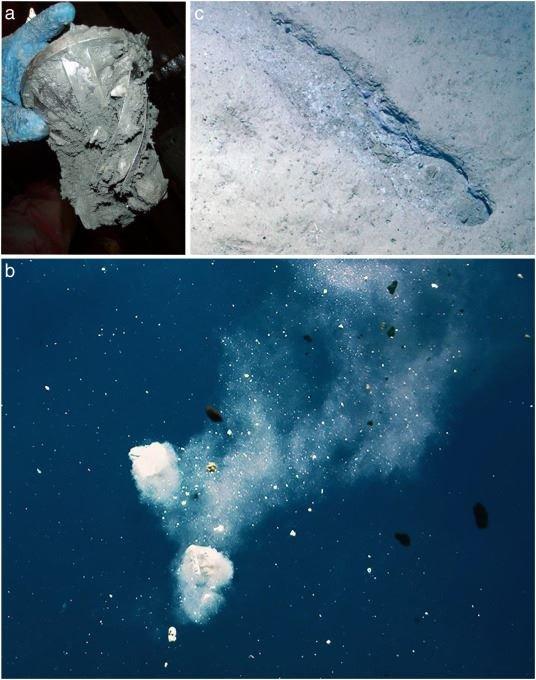
Looking even deeper into the subsurface however, pockmarks buried far beneath the seafloor are indicative of even longer term methane seepage, possibly beginning as long as 2.7 million years ago. 2.7 million years is quite an important age, especially around the Arctic, Svalbard and Barents Sea. 2.7 million years ago was the onset of Northern Hemisphere glaciations so likely the coinciding onset of seepage at the same time as glaciations in the Northern Hemisphere intensified are actually not a coincidence. It is very possible that when the load of ice on the crust got to a ‘critical’ level, faults and fractures might have been reactivated, dilated or similar leading to gas migration from the subsurface to the seafloor and resulting in methane seepage.
Relevant Literature
Bünz, S., Polyanov, S., Vadakkepuliyambatta, S., Consolaro, C., & Mienert, J. (2012). Active gas venting through hydrate-bearing sediments on the Vestnesa Ridge, offshore W-Svalbard. Marine geology, 332, 189-197.
Panieri, G., Bünz, S., Fornari, D. J., Escartin, J., Serov, P., Jansson, P., ... & Gracias, N. (2017). An integrated view of the methane system in the pockmarks at Vestnesa Ridge, 79 N. Marine Geology, 390, 282-300.
Pape, T., Buenz, S., Hong, W. L., Torres, M. E., Riedel, M., Panieri, G., ... & Bohrmann, G. (2020). Origin and transformation of light hydrocarbons ascending at an active pockmark on Vestnesa Ridge, Arctic Ocean. Journal of Geophysical Research: Solid Earth, 125(1), e2018JB016679.
Petersen, C. J., Bünz, S., Hustoft, S., Mienert, J., & Klaeschen, D. (2010). High-resolution P-Cable 3D seismic imaging of gas chimney structures in gas hydrated sediments of an Arctic sediment drift. Marine and Petroleum Geology, 27(9), 1981-1994.
Plaza‐Faverola, A., Bünz, S., Johnson, J. E., Chand, S., Knies, J., Mienert, J., & Franek, P. (2015). Role of tectonic stress in seepage evolution along the gas hydrate‐charged Vestnesa Ridge, Fram Strait. Geophysical Research Letters, 42(3), 733-742.
Plaza‐Faverola, A., Vadakkepuliyambatta, S., Hong, W. L., Mienert, J., Bünz, S., Chand, S., & Greinert, J. (2017). Bottom‐simulating reflector dynamics at Arctic thermogenic gas provinces: An example from Vestnesa Ridge, offshore west Svalbard. Journal of Geophysical Research: Solid Earth, 122(6), 4089-4105.
Schneider, A., Panieri, G., Lepland, A., Consolaro, C., Crémière, A., Forwick, M., ... & Knies, J. (2018). Methane seepage at Vestnesa Ridge (NW svalbard) since the last glacial maximum. Quaternary Science Reviews, 193, 98-117.
Smith, A. J., Mienert, J., Bünz, S., & Greinert, J. (2014). Thermogenic methane injection via bubble transport into the upper Arctic Ocean from the hydrate‐charged Vestnesa Ridge, Svalbard. Geochemistry, Geophysics, Geosystems, 15(5), 1945-1959.
Vogt, P. R., Crane, K., Sundvor, E., Max, M. D., & Pfirman, S. L. (1994). Methane-generated (?) pockmarks on young, thickly sedimented oceanic crust in the Arctic: Vestnesa ridge, Fram strait. Geology, 22(3), 255-258.
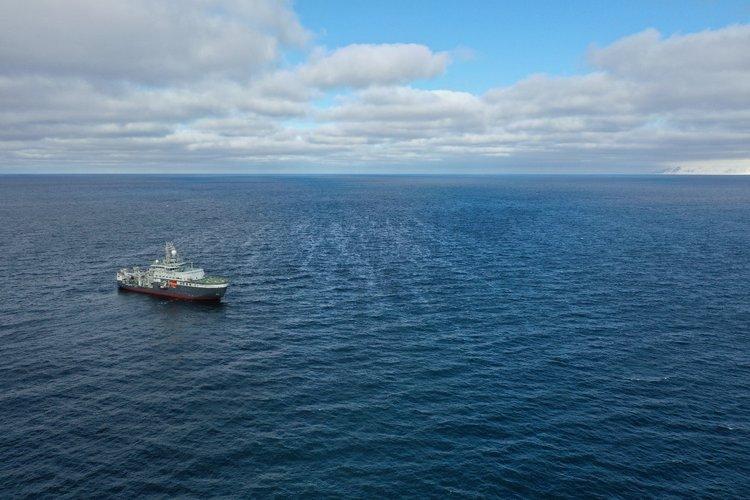
Prins Karls Forland is a long narrow island off the western coast of Svalbard, which was first sighted by Willem Barentsz in 1596. But our study site ‘Prins Karls Forland’ is actually a little bit offshore from the actual Prins Karls Forland. We are interested in the general offshore West Svalbard area because of the immense amount of methane seep sites around, but one specific site offshore Prins Karls Forland also seeps oil. The area shares a similar geology and geologic history to Svalbard and there is a lot of evidence of hydrocarbon from Svalbard - primarily the coal deposits that make up a significant part of Svalbard’s history. The Barents Sea and Svalbard regions have undergone many of the same uplifts and subsidence, erosion and depositions - so hydrocarbons in varying forms are to be expected.
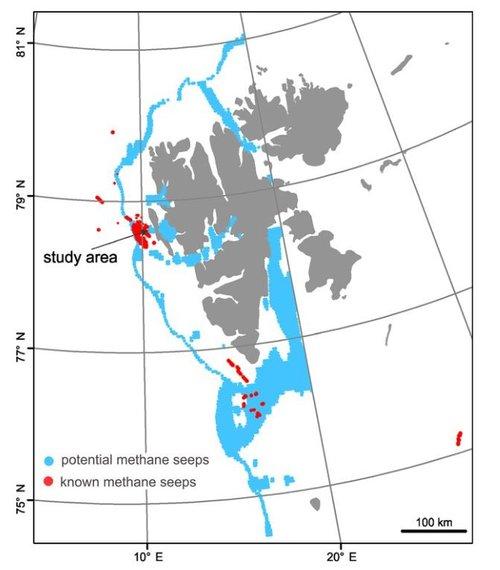
What attracted us to studying this area, is that the oil seep is actually visible on the surface of the ocean. The oil slick on the surface is clear from standing on the research vessel and even clearer just some meters higher using a drone. When we first dove with the ROV Ægir at this site, small black oil bubbles were an immediate contrast to the white-blue bacterial mats. While we do not yet know exactly what rocks beneath the seafloor are supplying the oil, or the precise way oil is getting to the seafloor we do know that the other methane seep sites offshore Prins Karls Forland have no documented oil seepage alongside the methane seep.
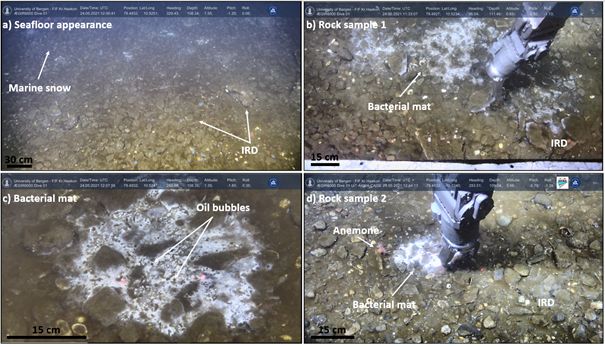
Franek, P., Plaza‐Faverola, A., Mienert, J., Buenz, S., Ferré, B., & Hubbard, A. (2017). Microseismicity linked to gas migration and leakage on the Western Svalbard Shelf. Geochemistry, Geophysics, Geosystems, 18(12), 4623-4645.
Gabrielsen, R. H., Kløvjan, O. S., Haugsbø, H., Midbøe, P. S., Nøttvedt, A., Rasmussen, E., & Skott, P. H. (1992). A structural outline of Forlandsundet Graben, Prins Karls Forland, Svalbard. Norsk Geologisk Tidsskrift, 72(1), 105-120.
Gründger, F., Probandt, D., Knittel, K., Carrier, V., Kalenitchenko, D., Silyakova, A., ... & Niemann, H. (2021). Seasonal shifts of microbial methane oxidation in Arctic shelf waters above gas seeps. Limnology and Oceanography, 66(5), 1896-1914.
Moser, M., Bergès, B., & Ferré, B. (2019, January). Long-term multibeam monitoring of natural methane seepage offshore Prins Karls Foreland, Svalbard. In Geophysical Research Abstracts (Vol. 21).
Myhre, C. L., Ferré, B., Platt, S. M., Silyakova, A., Hermansen, O., Allen, G., ... & Mienert, J. (2016). Extensive release of methane from Arctic seabed west of Svalbard during summer 2014 does not influence the atmosphere. Geophysical Research Letters, 43(9), 4624-4631.
Panieri, G., Graves, C. A., & James, R. H. (2016). Paleo‐methane emissions recorded in foraminifera near the landward limit of the gas hydrate stability zone offshore western Svalbard. Geochemistry, Geophysics, Geosystems, 17(2), 521-537.
Portnov, A., Vadakkepuliyambatta, S., Mienert, J., & Hubbard, A. (2016). Ice-sheet-driven methane storage and release in the Arctic. Nature communications, 7(1), 1-7.
Veloso‐Alarcón, M. E., Jansson, P., De Batist, M., Minshull, T. A., Westbrook, G. K., Pälike, H., ... & Greinert, J. (2019). Variability of acoustically evidenced methane bubble emissions offshore western Svalbard. Geophysical Research Letters, 46(15), 9072-9081.
Wallmann, K., Riedel, M., Hong, W. L., Patton, H., Hubbard, A., Pape, T., ... & Bohrmann, G. (2018). Gas hydrate dissociation off Svalbard induced by isostatic rebound rather than global warming. Nature communications, 9(1), 1-9.
Ingøydjupet is a trough in the Barents Sea formed by glaciers stretching out from Norway northwards into the Barents Sea. The area formed a very long time ago, when the rocks that make up the Barents Sea were a lot further south - around the equator actually. some 400 million years ago, the tectonic event that formed the mountains of Norway started. This event is called the Caledonian Orogeny - an orogeny is a mountain building event in geology terms. Later on, the tectonic regime reversed itself, from building mountains and uplifting to stretching apart and subsiding. During the various subsidence episodes a great many approximately north-south or north east - south west oriented faults were active and formed a series of basins and highs. As in the rest of the Barents Sea, the gradual subsidence from dry land to below the sea led to a great deal of organic matter deposition in the basins underlying the Ingøydjupet trough.
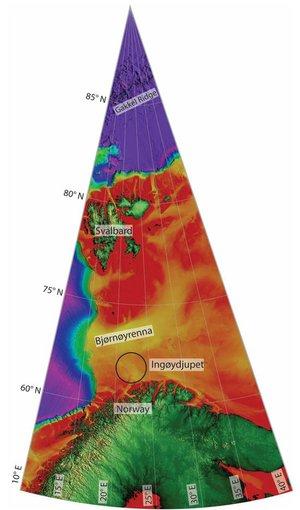
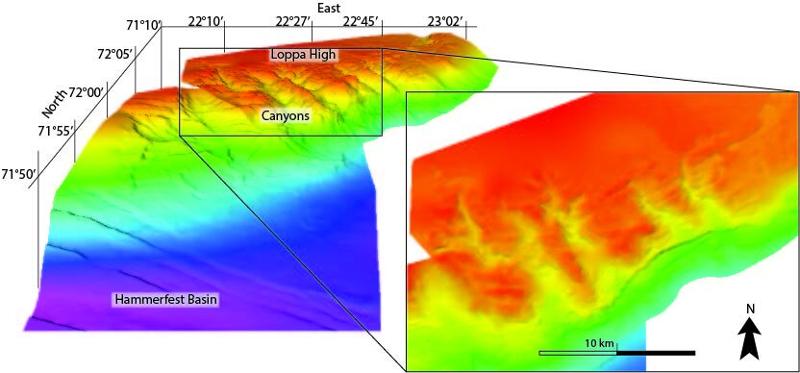
A common observation in the Barents Sea is that methane seepage was a lot stronger right at the end of the glaciations (about 10,000 years ago, depending on the location in the Barents Sea). That seepage has been getting less intense over time according to multiple scientific investigations. We know that ice and the Barents Sea are intrinsic but we don’t yet have all the details of how ice and the fluid flow systems of the Barents Sea have interacted through time and a major question at the Ingøydjupet study site is to try and untangle interactions between subsurface fluid and ice.
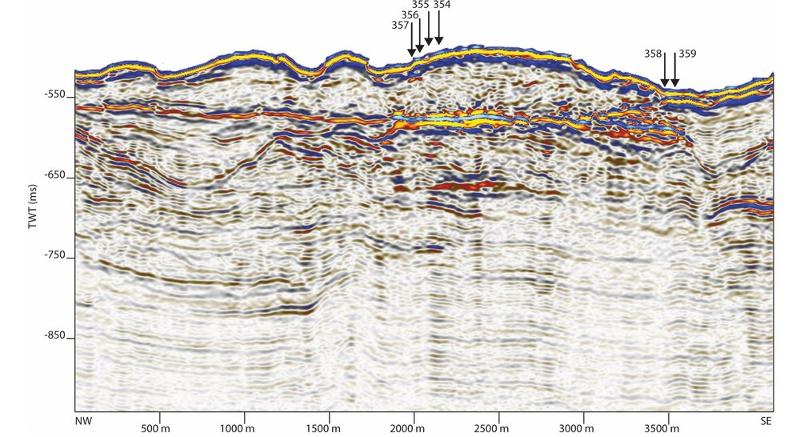
Related Literature
Andreassen, K., Laberg, J. S., & Vorren, T. O. (2008). Seafloor geomorphology of the SW Barents Sea and its glaci-dynamic implications. Geomorphology, 97(1-2), 157-177.
Dijkstra, N., Junttila, J., Husum, K., Carroll, J., & Hald, M. (2015). Natural variability of benthic foraminiferal assemblages and metal concentrations during the last 150 years in the Ingøydjupet trough, SW Barents Sea. Marine Micropaleontology, 121, 16-31.
Duran, E. R., di Primio, R., Anka, Z., Stoddart, D., & Horsfield, B. (2013). 3D-basin modelling of the Hammerfest Basin (southwestern Barents Sea): A quantitative assessment of petroleum generation, migration and leakage. Marine and petroleum geology, 45, 281-303.
Duran, E. R., di Primio, R., Anka, Z., Stoddart, D., & Horsfield, B. (2013). Petroleum system analysis of the Hammerfest Basin (southwestern Barents Sea): Comparison of basin modelling and geochemical data. Organic geochemistry, 63, 105-121.
Jensen, H. K., Boitsov, S., Finne, T. E., Klungsøyr, J., & Knies, J. (2009). Physical and chemical traces of anthropogenic influence at the seabed and in the sediments in Ingøydjupet, Southern Barents Sea. Norwegian Journal of Geology/Norsk Geologisk Forening, 89.
Junttila, J., Carroll, J., Husum, K., & Dijkstra, N. (2014). Sediment transport and deposition in the Ingøydjupet trough, SW Barents Sea. Continental Shelf Research, 76, 53-63.
Sattar, N., Juhlin, C., Koyi, H., & Ahmad, N. (2017). Seismic stratigraphy and hydrocarbon prospectivity of the Lower Cretaceous Knurr Sandstone lobes along the southern margin of Loppa High, Hammerfest Basin, Barents Sea. Marine and Petroleum Geology, 85, 54-69.
Leirdjupet Fault Complex is located in Bjørnøyrenna/the Bear Island Trough in the Barents Sea. Bjørnøyrenna was carved out due to ice streams during the northern hemisphere glaciations, so the seafloor is full of marks related to ice activity like ploughmarks and glacial lineations. Along the northern margin of Bjørnøyrenna, the warm and salty Atlantic water transported by the North Cape Current meets the chilly Arctic waters flowing south. This meeting of waters defines the Arctic Front and the (modern) marginal ice zone. The marginal ice zone marks the southward extent of the ice edge during winter under present day conditions. CAGE expeditions show significant natural methane seepage along the Leirdjupet Fault Complex.
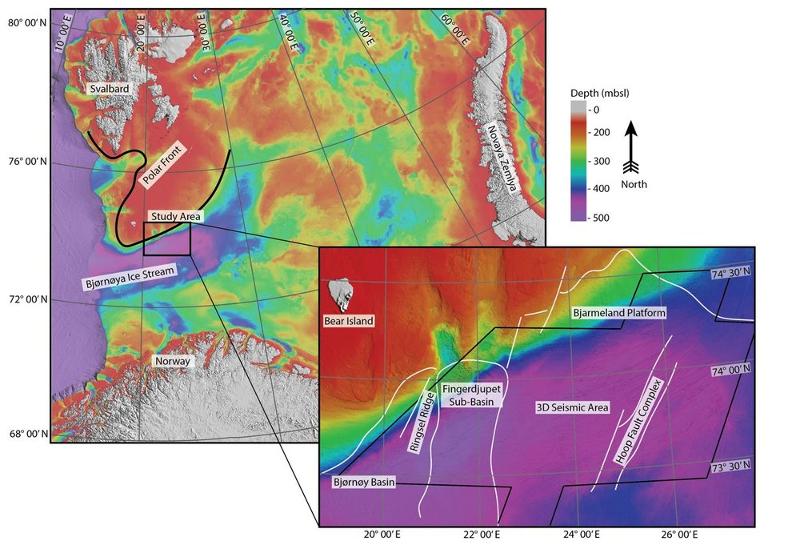
The Barents Sea has a long and complicated geologic history, with multiple opposing tectonic events, uplifts, subsidences and ultimately a lot of erosion during the glaciation events in the more recent past. Ultimately, many basins, highs and fault complexes throughout the Barents Sea have formed and in the area of the Leirdjupet Fault Complex these are primarily oriented NE-SW and N-S. Another important thing to consider in the Barents Sea is that there have been many times throughout history where the Barents Sea was much shallower than it is today, in fact at times it was dry land. Ultimately, the Barents Sea ended up having a lot of organic matter deposited into its basins throughout its history. This organic matter got buried, and became hydrocarbon. Nowadays, that hydrocarbon is leaking out of the Leirdjupet Fault Complex and we are able to investigate the dynamics of methane seepage here. Some of our major aims at this site are to understand the role in glaciations and ice in the fluid flow system, and to understand how the Leirdjupet Fault Complex came to be one of the largest natural methane seep areas in the SW Barents Sea.
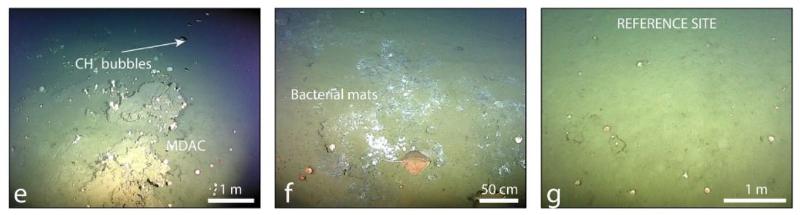
Relevant Literature
Argentino, C., Waghorn, K. A., Vadakkepuliyambatta, S., Polteau, S., Bünz, S., & Panieri, G. (2021). Dynamic and history of methane seepage in the SW Barents Sea: new insights from Leirdjupet Fault Complex. Scientific reports, 11(1), 1-13.
Faleide, J.I., Tsikalas, F., Mjelde, R., Wilson, J. & Eldholm, O. (2008). Structure and evolution of the continental margin off Norway and the Barents Sea. Episodes, 31, 82-91
Gernigon, L., Brönner, M., Roberts, D., Olesen, O., Nasuti, A. & Yamasaki, T. (2014). Crustal and basin evolution of the southwestern barents sea: from caledonian orogeny to continental breakup. Tectonics, 44(4), 347-373.
Indrevær, K., Gabrielsen, R.H. & Faleide, J.I. (2017). Early Cretaceaous synrift uplift and tectonic inversion in the Loppa High area, Southwestern Barents Sea, Norwegian Shelf. Journal of the geological Society, 174(2), 242-254.
Haarpaintner, J., Gascard, J. C., & Haugan, P. M. (2001). Ice production and brine formation in Storfjorden, Svalbard. Journal of Geophysical Research: Oceans, 106(C7), 14001-14013.
Loeng, H. (1991). Features of the physical oceanographic conditions of the Barents Sea. Polar research, 10(1), 5-18.
Loeng, H., Ozhigin, V., & Ådlandsvik, B. (1997). Water fluxes through the Barents Sea. ICES Journal of Marine Science, 54(3), 310-317.Ingvaldsen, R. B. (2005). Width of the North Cape Current and location of the Polar Front in the western Barents Sea. Geophysical Research Letters, 32(16).
Serck, C.S., Faleide, J.I., Braathen, A., Kjølhamar, B. & Escalona, A. (2017). Jurassic to Early Cretaceous basin configuration(s) in the Fingerdjupet Subbasin, SW Barents Sea. Marine and Petroleum Geology, 86, 874-891.
Winsborrow, M. C., Andreassen, K., Corner, G. D., & Laberg, J. S. (2010). Deglaciation of a marine-based ice sheet: Late Weichselian palaeo-ice dynamics and retreat in the southern Barents Sea reconstructed from onshore and offshore glacial geomorphology. Quaternary Science Reviews, 29(3-4), 424-442.